Battery
A satellite in its orbit tries to maximise the power intake, while also balancing the subsystem requirements. However, this might not always provide enough power required for all of the functioning. This is where batteries along with its different types come in.
Batteries (mostly rechargeable in satellites) are commonly used to provide power:
- during launch and post-launch until the deployment of solar panels (if the solar panels are deployable)
- to the spacecraft, its equipment and payload during Sun eclipse periods
- for communication and data transmission
- to keep the electronics within a specified temperature range
You might already be knowing that these batteries must be capable of withstanding severe launch environments (vibration, shock, acceleration) along with tolerance to high intensity radiations. Hence they should provide maximum electrical energy in minimum weight and volume. The most stringent requirement is maintaining the temperature of the battery.
In general, batteries that display higher gravimetric(higher mass) and volumetric energy densities(higher volume) can manifest in reduced mass and volume for the power subsystem and thus increased payload and mission capabilities. Batteries with tolerance to extreme environment can, on the other hand, be mission enabling and may facilitate increased scientific capabilities of the mission. [1]
Contents
Factors affecting battery performance
Capacity
Capacity is adversely affected by high storage and high and low operation temperatures than cell optimum temperature, higher discharge rates, the stand time between charge and discharge. State of charge, the extent to which the battery is charged, opposite to Depth of Discharge, should also not be kept very high during storage.
Voltage
The voltage of the cell decreases during the discharge, and the shape of the discharge voltage curve is affected by temperature, discharge rate, cycle life, service life, and the electrochemical reactions occurring within the cell. The voltage typically is lower and decreases faster with increased discharge rates and longer cycle life.
Discharge Current Rate
Decreased capacity, voltage, and life and increased IR losses and heating are seen with higher discharge current rates, along with a more rapid decrease in voltage during the discharge. If we discharge keeping cutoff voltage constant, lower current rate would have additional capacity available, above that cutoff voltage, than higher one. Discharge rates are commonly specified as multiples of the C rate, which is the current that will discharge the battery to the cutoff voltage in one hour. 1C rate means battery will be discharged completely in 1 hr by the discharge current.
Charge Current Rate
Less capacity is restored and increased heating occurs when higher charge current rates are used. The magnitudes of the capacity decrease are temperature dependent. When a cell is charged at a higher current rate to the end-of-charge voltage, more . To add more capacity below the cutoff voltage, lower rate is preferred. Charge rates are also commonly specified as multiples of the C rate.
Continuous or Intermittent Discharge
When a battery rests after discharge, voltage recovery happens due to certain physical and chemical changes happening. Thus, the voltage of a battery that has dropped during a high-rate discharge will rise after a rest period. This improvement is generally greater after discharge at higher currents and also is dependent on the end-of-discharge voltage, temperature, and the length of the rest period.
Methods of charging
Constant current, constant voltage, and constant current-constant voltage, explained later.
Temperature
Lower temperatures lead to a reduction in chemical activity and increase in internal resistance of battery. On the contrary, batteries exposed to hotter average temperatures lose their ability to store energy. The optimum temperature is dependent on the specific battery chemistry and design and can be tailored somewhat. For most Li-ion batteries the optimum temperature is between 20 and 40°C. The cell’s capacity falls off most rapidly with increasing discharge load and decreasing temperature. Discharging at high rates could cause anomalous effects as the battery may heat up to temperatures far above ambient.
Charging Voltage and Voltage Regulation
Allowing the lowest possible end-of-discharge voltage and the widest voltage range leads to the highest available capacity. A voltage regulator can be used to convert the varying output voltage of the battery into a constant output voltage consistent with the equipment requirements. This allows the full capacity of the battery to be used; the only tradeoff is that the voltage regulator has losses.
Vibration and Shock
These can cause internal shorts that might lead to a mishaps like venting of electrolyte, possible fire and fracture of cell case.
Cycle Life
Number of charge and discharge cycles performed by the battery lowers both its voltage and capacity available on discharge.
Battery Charging
Depending on the requirement, a battery may be discharged using constant current, constant resistance, or constant power loads, or variable loads. The time that the battery will deliver the required capacity is inversely proportional to the average current.
Constant Current Charging
This is one of the most effective ways to charge many different types of batteries. In this mode, current is set so that that the power output at the end-of-discharge voltage is equal to the minimum level required. The average current drain on the battery is lower and the discharge time is longer. Charge times are relatively long with the disadvantage that the battery may overheat if it is overcharged, leading to premature battery replacement. This method is suitable for Ni-MH type of batteries. The battery must be disconnected or a timer function with charging. [2]
Constant Voltage Charging
A constant voltage charge provides a high initial charge to the battery then it slowly decreases until the voltage level is read again, at which point the battery charge will again be raised and slowly lowered again. The constant charging of the battery with this method will cease once the full voltage of the battery is reached.[3] The battery can be left connected to the charger until ready for use and will remain at that “float voltage”, trickle charging to compensate for normal battery self-discharge. This method enables fast charging rates and is suitable for lead acid types, but not for Nickel Metal Hydride (Ni-MH) or Lithium-Ion (Li-ion) types. [2]
Constant Current Constant Voltage (CC-CV) Charging
In this method, battery is charged with a constant current until voltage reaches the end-of-charge(EOC) voltage. Thereafter, charger switches to a constant voltage charging, where the charging current falls with time. The battery is said to be charged when the charging current drops to a predetermined limit. This is the preferred mode for charging Li-ion batteries.
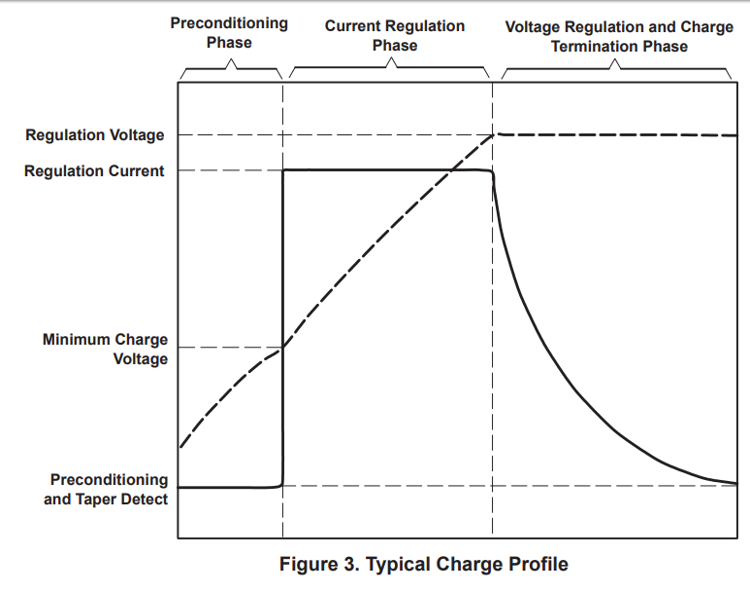
Battery Discharging
Depth of Discharge
It is the capacity that is discharged from a fully charged battery, divided by battery nominal capacity. Example: if a 100Ah battery is discharged for 20 minutes at 50A, the Depth of Discharge is: 50*(20/60)/100= 16.7%
Depth-of-Discharge (DoD) (%) = [E(Ah) removed/ E(Ah) rated]*100
State of Charge = 100- Depth of Discharge
Battery Management System
Three main objectives common to all battery management systems:
- Protect the cells or the battery from damage
- Prolong the life of the battery
- Maintain the battery in a state in which it can fulfil the functional requirements of the application for which it was specified.
To achieve them BMS may incorporate one or more of the following functions:
- Cell Protection
- Charge Control: More batteries are damaged by inappropriate charging than by any other cause.
- Demand Management: Its objective is to minimise the current drain on the battery by designing power saving techniques into the applied circuitry and thus prolong the time between battery charges.
- SOC (State of Charge) Determination: This may simply be for providing the user with an indication of the capacity left in the battery, or it could be needed in a control circuit to ensure optimum control of the charging process.
- SOH (State of Health) Determination: It is a measure of a battery's capability to deliver its specified output. This is vital for assessing the readiness of emergency power equipment and is an indicator of whether maintenance actions are needed.
- Cell balancing: In multi-cell battery chains small differences between cells due to production tolerances or operating conditions tend to be magnified with each charge / discharge cycle. Weaker cells become overstressed during charging causing them to become even weaker, until they eventually fail causing premature failure of the battery. Cell balancing is a way of compensating for weaker cells by equalising the charge on all the cells in the chain and thus extending battery life.
- History: Monitoring and storing the battery's history is another possible function of the BMS. This is needed in order to estimate the State of Health of the battery, but also to determine whether it has been subject to abuse. This does not have to necessarily be stored, it can as well be transmitted to the ground station.
- Communication: Most BMS systems incorporate some form of communication between the battery and the charger or test equipment. Communication interfaces are also needed to allow the user access to the battery for modifying the BMS control parameters or for diagnostics and test.
Information about Available Batteries
Silver-Zinc batteries
The earliest use of a battery in an orbital spacecraft was the primary Ag-Zn battery used in the Russian spacecraft, Sputnik, launched October 4, 1956. This primary battery was used to provide power for communication and spacecraft operation. There were no solar cells available for charging, and thus when the energy was depleted, communication was terminated.
These have been used in several US spacecrafts.
Nickel-Cadmium Batteries
Intended for use where the battery will experience frequent charge/discharge cycles. Advantages include its low cost, flat discharge rate, its capacity to withstand deep discharges without damaging the cells and to remain sturdy for rough environments.
These have been successfully applied to LEO(low-earth orbit) microsatellite and minisatellite programs. It has performed some 70,000 charge/discharge cycles over a 14 year period and is still useful.
Disadvantages could be the memory effect (the condition that battery loses capacity to hold maximum energy when repeatedly overcharged after partial discharging) and its relatively low energy density compared with newer battery systems.
The “super” Ni-Cd cells, developed by Eagle-Picher, were the next step to enhance the Ni-Cd energy density and life. NASA utilized these in the late 80s on small satellites, such as the small Explorer series.
Nickel-Hydrogen Batteries
These have found wide use in high-reliability space applications that require extended service life. Replacement of Cd with hydrogen reduced weight and increased energy thus almost doubling the specific energy. The cells often appear capable of handling the stresses of inadvertent reversal or excessive overcharge with little evidence of the damage or performance degradation that had been the rule for nickel-cadmium cells. [4]
Li-ion Batteries
High energy density, high specific energy and long cycle life make Li-ion batteries promising power sources for satellites. It offers significant advantages in terms of mass(almost half of nickel hydrogen batteries for the same stored energy), volume and temperature range. The batteries also display good tolerance to occasional deep discharge. Li-Ion cells also display impressive and adequate tolerance to radiation levels as high as 18 Mrad (1 rad=0.01 J/kg ; dose causing 100 ergs of energy to be absorbed by 1 gram of matter) and exhibit a loss of less than 10 % upon such high levels of radiation exposure. Furthermore, a portion of this loss can be attributed to the cycling or storage during this incremental radiation exposure. [1]
Significant advances have been made in the cathode materials and electrolytes for Li-ion cells and batteries. Several new cathodes with high specific capacity approaching 250 mAh/g, coupled with high voltage and improved thermal stability have been identified. Likewise, several new electrolytes for enabling operations at -60 °C have been demonstrated. These advances are expected to results in advanced lithium-ion cells and batteries with high specific energy and wide range of operating temperatures, as desired in future space missions. [1] However, the polymer li-ion cells have an additional problem with electrolyte leakage under abusive conditions.[5]
Comparison between the batteries
System | Specific Energy (Wh/kg) | Energy Density (W/L) | Operating Temperature (°C) | Lifetime (in Years) | Cycle life (* @partial Depth of Discharge) |
Silver-Zinc | 100 | 200 | -10 to +25 | <1 | <100 |
Nickel Cadmium | 35 | 100 | -10 to 25 | >5 | >30,000* |
Nickel-Hydrogen | 40 | 80 | -10 to 30 | 5-10 | >40,000* |
Li-ion | 100 | 240 | -30 to 40 | 4 | 1000 |
Traditionally used batteries use aqueous electrolyte (eg. Ni-Cd, Ni-H2, lead-acid) mainly because water is the best and cheapest chemical solvent for most ionic compounds and such solutions can have very good ionic conductivity. However water decomposes at a relatively low voltage limiting the cell voltage of aqueous cells to a maximum in the region of 1.5 to 2.0 V. For satellites requiring more than 3 years of operation, batteries used must achieve 1000 to 33000 cycles without the requirement of maintenance. This is considerably in excess of the cycle lives demanded by most terrestrial battery applications and it is the cycle life requirement that has confined spacecrafts to the use of well-proven alkaline battery technologies- Ni-Cd and Ni-H2. However, when used to 80% of their available capacity, these technologies offer useful mass energy densities of no more than 24 and 36 watt-hours/kg respectively at battery level( taking into account mass of battery packaging). Provided life-cycle requirements can be met, lithium ion technology provided for better in terms of cost/performance factor.[6]
Hazards and Prevention
Risk regarding batteries are broadly due to: [5]
- Overcharging
- Over-discharge
- External and internal short circuits
- High temperatures - ambient as well as cell temperature limit
- Under voltage- Exceeding depth of discharge (DOD) limits
- Pressure build up in the cell
The individual cells should be able to survive a short circuit with an opening to release gaseous products, to prevent pressure build-up in the cell . Circuit should be designed keeping overcharge, over-discharge, short circuits and temperature considerations in mind. Battery designing must have a significant safety factor.
Lithium cells shouldn't be kept on conducting surfaces unless they have appropriate conduction protection. Spot welding, and not soldering, should be used to attach leads directly to a cell.[5] Caution also has to be taken against radiation - via either MLI (Multi-Layer Insulation) or a black coating. Internal short prevention in lithium-ion batteries effectively opens the battery circuit, shutting off current flow through the battery.
A PTC (Positive Temperature Coefficient) switch can also be used to prevent short-circuiting by inhibiting high currents. As temperature increases above a limit, material resistance faces a large increase and being reversible they cycle back to conductive state when we have normal condition again.
CIDs (Current Interrupter Devices) can prevent further charging of a battery until internal pressure(of battery) is alleviated. These devices prevent venting of hazardous electrolytes and bursting due to buildup of high pressures.[7] However, both PTCs and CIDs may fail when exposed to high voltages due to other failures. The use of bypass diodes is recommended to prevent these failures.
The surfaces of battery terminals that extend inside the battery case need to be insulated with potting materials to prevent unintentional contact with other conductors inside the case and also to prevent bridging by electrolyte leaks. Wires inside the battery case should be insulated, restrained from contact with cell terminals, protected against chafing and physically constrained from movement due to vibration or bumping.[5]
Certification of the crimp is critical for ensuring that individual cells will not leak after launching.[7] Cell terminals need to be protected from contact with other conductive surfaces.
If you are done reading this page, you can go back to Electrical Subsystem
References
- ↑ 1.0 1.1 1.2 1.3 http://ieeexplore.ieee.org/stamp/stamp.jsp?arnumber=4161575
- ↑ 2.0 2.1 http://power-topics.blogspot.in/2016/05/constant-voltage-constant-current.html
- ↑ https://www.doityourself.com/stry/4-best-battery-charging-techniques
- ↑ http://aerospace.wpengine.netdna-cdn.com/wp-content/uploads/2012/03/bk_ni-hyd-batteries-prin-pract_ch1.pdf
- ↑ 5.0 5.1 5.2 5.3 https://ntrs.nasa.gov/archive/nasa/casi.ntrs.nasa.gov/20090023862.pdf
- ↑ http://articles.adsabs.harvard.edu/cgi-bin/nph-iarticle_query?bibcode=1998ESASP.416...17D&db_key=AST&page_ind=0&data_type=GIF&type=SCREEN_VIEW&classic=YES
- ↑ 7.0 7.1 https://ntrs.nasa.gov/archive/nasa/casi.ntrs.nasa.gov/20150020899.pdf